Producing Copper Nature's Way: Bioleaching
Copper Applications in Mining & Extraction
Summary | Examples | Background | Chemistry of Bioleaching | Microbiology of Bioleaching | Leaching Method | Costs | Research | Conclusions | References
Summary 
Bioleaching is the extraction of a metal from sulfide ores or concentrates using materials found native to the environment; namely, water, air and microorganisms. In other words, bioleaching is the commercialization of the ability of certain bacteria and archaea, found in nature, to catalyze the oxidation of sulfide minerals. It is the leaching of sulfide minerals that distinguishes bioleaching from conventional acid leaching wherein only oxidized minerals are leached.
An associated process is biooxidation. This is the oxidation of sulfide minerals associated with but not necessarily part of the mineral of interest to be extracted. Bioleaching is used today in commercial operations to process ores of copper, nickel, cobalt, zinc and uranium, whereas, biooxidation is used in gold processing and coal desulfurization.
Bioleaching involves the use of microorganisms to catalyze the oxidation of iron sulfides to create ferric sulfate and sulfuric acid. Ferric sulfate, which is a powerful oxidizing agent, then oxidizes the copper sulfide minerals and the copper contained is then leached by the sulfuric acid formed. In the case of uranium, the ferric sulfate oxidizes tetravalent uranium oxide, which is insoluble in acid, to hexavalent uranium oxide, which is then leached by the sulfuric acid. In biooxidation of refractory gold ores bacteria are used to oxidize, and therefore make soluble, an iron sulfide matrix in which the gold particles are imbedded and thus make the gold available for cyanide leaching. Likewise, in coal desulfurization, bacteria are used to oxidize the pyrite contaminant in the coal thus making the sulfur soluble as ferric sulfate.
Since bioleaching is a natural process, one undesirable effect is the creation of metalliferous acid drainage from the slow weathering of sulfide mineral outcrops and from abandoned mines. However, as in the case of Rio Tinto, in Spain, even this can be turned into an asset by recovery of the copper from such waters.
Examples of Current Industrial Bioleaching Operations 1 
- Acid Mine Drainage
- Rio Tinto, Spain
- Dump Leaching
- Bagdad, USA
- Morenci, USA
- Pinto Valley, USA
- Sierrita, USA
- Heap Leaching
- Cerro Colorado, Chile
- Cananea, Mexico
- Chuquicamata SBL, Chile
- Collahuasi, Chile
- Girilambone, Australia
- Ivan Zar, Chile
- Morenci, USA
- Punta del Cobre, Chile
- Quebrada Blanca, Chile
- Salvador QM, Chile
- Sociedad Minera Pudahuel, Chile
- Zaldívar, Chile
- Bioleaching of Gold Concentrates
- Ashanti, Ghana
- Fairview, Zambia
- Harbour Lights, Australia
- Mount Leyshon, Australia
- Sao Bento, Brazil
- Wiluna, Australia
- Youanmi, Australia
Following early development work and application in the United States, Chile has become a major developer of bioleaching on an industrial scale. In 2001, there were 13 companies using bioleaching and 30 companies in all using leach/SXEW technology. In 2001, bioleaching constituted approximately 10% of Chilean copper production and heap and dump leaching, in general, constituted approximately 30% of Chilean production. 2 The first Chilean plant to be put into operation was S.M. Pudahuel. The plant was initiated in 1982 using the Thin Layer Bacterial leach process (TLB) - a water saving process - and leaches copper oxides and secondary sulfide minerals.
Bioleach processing differs depending on the type of resources to be processed. It is used today in three different regimes dependent upon the raw material types to be processed:
- Dump leaching - waste rock, low grade ore or concentrator tailings (low grade, oxides and secondary sulfides) are leached where placed for disposal. (Mature technology, widely used)
- Heap leaching - newly mined run-of-the-mine (ROM) material (intermediate grade, oxides and secondary sulfides) is deposited in a heap on an impervious natural surface or a pad and leached. ROM may be leached as mined or may be partially crushed and mixed with acid prior to depositing on heap. (Mature technology, increasing use); Primary sulfides; i.e., chalcopyrite, leaching (Experimental only)
- Agitated leaching - intermediate- to high-grade, chalcopyrite concentrates are deposited in a tank and leached using mechanical agitation. (Experimental only)
The raw material type also determines the type of microorganisms involved:
- Waste dump leaching uses mesophilic (ambient temperature) microorganisms, i.e., bacteria.
- Heap leaching of ore may involve mesophilic or thermophilic (high temperature) microorganisms, i.e., archaea, depending on the mineral species present and the physical chemistry of the leach environment.
- Leaching of chalcopyrite and concentrate leaching requires thermophilic microorganisms. (Chalcopyrite can be leached using mesophilic bacteria; however, recovery is low and retention time is several years.)
Background 
The results of natural microbial leaching have been known since ancient times. Pliny the Elder (23-73 AD), who had a passion for observing the wonders of nature, discusses the " vitreolus quasi vitrum" - a glass-like substance - found on rocks in his treatise on natural history. 3 One of the earliest records of utilizing the effects of bioleaching is from the island of Cyprus. Galen, a Greek physician from Pergamum, in 162 A.D., is reported to have collected cuperiferous solutions from mine water from the mines of Skouriotissa and concentrated them by evaporation to form crystals of copper sulfate. 4 Recent findings have revealed evidence that predates this account. 5 Indeed, observations have been made on the natural leaching of copper and the formation of "gall springs" during the East Han Dynasty (206 BC-220 AD) in China. The "Gall-Copper Process" was recorded as being used during the Song Dynasty (960-1271AD). Copper was precipitated from solution by dipping iron into the blue vitriol solution - a process identified as early as 150 BC in China. 6 Therefore, presumably, the recognition of a natural copper leaching process can be identified as early as that date.
Iron-rich acidic waters draining from abandoned coal and metal mines as well as from unmined mineralized areas are another evidence of microbial leaching. In fact, history records that mine water problems began at the same time that mining activities began. For example, in the area of the Iberian Pyritic Belt, exploited since prehistoric times, the production of acid mine drainage gave names to rivers like Tinto, Tintillo, Aguas Teñidas, referring to the river's characteristic color. In fact, at Rio Tinto, in Spain, 17th-century records describe the occurrence of copper-bearing waters. Formal microbial leaching continued at the Rio Tinto mine until the late 1970s. The UK-based mining company, Rio Tinto, which was formed in 1873, owes its name to these copper-bearing waters.
It was not until 1947 that these phenomena were attributed to bacteria. 7 Once identified, however, rapid steps were taken to commercialize the process. Commercial application of bacterial leaching began in the late 1950s at the Kennecott Utah Copper Company's Bingham Canyon Mine near Salt Lake City, Utah where it was observed that blue copper-containing solutions were running out of waste piles that contained copper sulfide minerals - something that should not have happened in the absence of powerful oxidizing agents and acid. Investigation revealed that naturally occurring bacteria were oxidizing iron sulfides in the piles, and the resulting ferric sulfate and sulfuric acid was acting as an oxidizer and leachant for copper sulfides. 8 These bacteria were given the name ferrooxidans for their ability to oxidize iron sulfides. A second set of bacteria was also identified and given the name thiooxidans for their ability to oxidize sulfur to yield sulfuric acid. The bacteria, which were native to the soil, in effect created a completely natural metallurgical processing plant.
Until recently, conventional acid-leaching of copper was utilized as a means of recovering copper from low-grade materials such as waste rock and flotation tailings and was conducted in dumps that were already in place. At least five factors have impeded the acceptance of leaching as a major commercial process:
- The lack of an efficient way of recovering metallic copper from solutions.
- The very slow rate of leaching.
- The inability to process sulfide mineral concentrates.
- The inability to process chalcopyrite - the most abundant and, thus, the most economically important copper mineral.
- The inability to recover precious metals
Solutions are now in sight to rectify most of these impediments.
Prior to the development of the solvent extraction-electrowinning (SX/EW) process in 1959 (see Copper the Green Metal ), the only way to recover copper from an acid solution was by a process called cementation. In this process, copper was in effect "traded" for iron by contacting the copper-bearing solution with scrap iron. Impure metallic copper was precipitated on the iron surface while an electrochemical equivalent of iron went into solution. The resulting cement copper was then processed in a smelter and electrolytic refinery to produce high-purity copper. By the use of the SX/EW process, copper can now be extracted away from the iron and other impurities in solution and further purified and reduced to metallic metal by electrolysis without ever having to be melted.
Further, through the use of biooxidation, it is now possible to leach many of the copper sulfide minerals present in the ore. While chalcopyrite remains refractory to ambient temperature bioleaching, other copper sulfide minerals - the secondary sulfide minerals - such as chalcocite, covellite, bornite, etc. - are leachable by today's technology.
It is well known that increasing the temperature can increase the rate of a chemical reaction. However, microorganisms are sensitive to temperature and, until recently when new strains of temperature-resistant microorganisms ( thermophilic) were introduced, the reaction rate of bioleaching was too slow to consider conducting the process as a primary copper extraction process.
A number of companies have developed biooxidation processes for use in metal extraction. Some of these have found commercial application while others are still in the experimental or pilot plant stages:
Newmont Mining | BIOPRO™ Process - heap leaching of refractory gold ores |
---|---|
Gold Fields, Ltd | BIOX™ Process - agitated tank oxidation of refractory gold ores |
BHP Billiton, Ltd. 9 | BioCOP™ Process- agitated tank oxidation and leaching of copper sulfides BioNIC™ Process - agitated tank oxidation and leaching of nickel sulfides BioZINC™ Process - agitated tank oxidation and leaching of zinc sulfides |
BacTech Enviromet 10, 11 | BacTech/Mintek Process - agitated tank oxidation and leaching of copper sulfides |
GeoBiotics, Inc. 12, 13 | GEOCOAT™ Process - heap leaching sulfide mineral concentrates |
BacTech Enviromet Corp., in conjunction with Mintek, 14 has developed proprietary technologies for the high temperature leaching of copper concentrates. Working in conjunction with Industrias Penoles SA de CV, one of Mexico's largest and most diverse mining companies, they operated a 2.2-metric tons per day (mt/d) stirred-tank copper-concentrate bioleach demonstration plant in Monterrey, Mexico during 2001. A decision will be made in late 2002 regarding construction of a plant capable of producing 25,000 metric tons of cathode copper per year. 15
BHP Billiton and Codelco, in a joint venture, Alliance Copper Ltd., are constructing a demonstration plant at Chuquicamata, in northern Chile, that will produce 20,000 tons of cathodes a year starting in 2003 using Billiton's patented BioCOP™ process. 16 One purpose of the project is to process "dirty concentrates," i.e., those containing high levels of arsenic (enargite, Cu 3AsS 4) that are not desirable to be processed by smelting for environmental reasons. 17
Chemistry of Bioleaching 
The majority of copper minerals are sulfides, with chalcopyrite (CuFeS2) being the most abundant and thus economically the most important. Others of economic importance are bornite (Cu 5FeS 4), chalcocite (Cu 2S) and covellite (CuS). Sulfide minerals are insoluble in water or acid solutions unless they are first oxidized. While exposure to air is sufficient to oxidize these minerals the process is slow and inefficient. The kinetics of the oxidation process are vastly improved by the introduction of Thiobacillus ferrooxan and Thiobacillus thiooxan bacteria to the system. The former catalyzes the oxidation of iron whereas the latter catalyzes the oxidation of sulfur. 18
There are two dominant views on the mechanisms involved in bioleaching. The first is that the overall leaching process occurs by the microbial oxidation of ferrous to ferric ions followed by the chemical oxidation of the sulfide mineral by the ferric ion. This is known as the indirect mechanism. The second view envisages the microbial catalysis of the overall dissolution of the mineral. It has been proposed that the microorganisms interact with the mineral directly, enhancing the rate of oxidation, over and above that achieved by chemical oxidation. This is known as the direct mechanism. Regardless of the mechanism, it is the enhanced oxidation of the mineral created by the microorganism that creates the acid-solubility required for leaching to take place.
The overall chemical reactions for chalcopyrite are:
4CuFeS 2 + 11O 2 + 6H 2O Thiobacillus ferrooxin >> 4CuSO 4 + 4Fe(OH) 3 + 4S (1)
2S + 3O 2 + 2H 2O Thiobacillus thiooxan >> 2H 2SO 4 (2)
It should be noted that one of the problems that has had to be overcome in the leaching of chalcopyrite was that, when using natural bacteria, a passivating layer forms on the mineral surfaces that prevents further leaching. This passivating layer could come from one or both of two sources: (1) Fe(OH) 3 tends to form jarosite (KFe 3(SO 4) 2(OH) 6), which coats the unreacted material and forms a passivating layer and/or, (2) the elemental sulfur formed in reaction (1), which also tends to coat the surface. Covelite and chalcocite are much easier to leach. It has been found from fundamental electrochemistry that the newer technology, using thermophilic microorganisms such that the temperature can be raised to 60°C (140°F) or higher, destabilizes the passivating layer on chalcopyrite.
Microbiology of Bioleaching 
As indicated above, the original bacteria used in bioleaching were those that were native to the site where the leaching took place. However, once bioleaching gained acceptance as a potentially viable method for the recovery of metals from low-grade ores, flotation tailings and waste materials, efforts were undertaken to optimize the bacteria for the operation intended. "Native" bacteria, sometimes termed mesophilic bacteria, are sensitive to elevated temperatures and to some of the metals being extracted. Thus, microorganisms were sought that can withstand higher temperatures, thermophilic (and even hyperthermophilic; i.e., 60°C or higher) microorganisms, and are tolerant to the metals being extracted. One of the first locations to be investigated for suitable thermophilic bacteria was the hot springs at Yellowstone National Park. Others have been a hot spring in Iceland, a coalmine in Western Australia, a volcano in Italy and a slagheap in Germany.
Out of these collections have come starting cultures for a number of bacteria strains. For example, some bacteria in use today are: Acidianus brierleyi ( Sulfolobus brierleyi ), an aerobic, extremely acidophilic, thermophilic sulfur-metabolizing archaebacteria useful to 70°C (158°F); that was developed from a Yellowstone hot spring; 19 Acidianus infernos, useful to 88°C (190°F), that was developed from mud from the crater of the Solfatara volcano in Italy; 20 Sulfolobus metallicus, useful to 65°C (149°F), that was isolated from the lava field of the same volcano; 21 Metallosphaera sedula, useful to 65°C, that was isolated from a thermal spring; 22 Metallosphaera prunae, useful to 80°C (176°F), that was found in the smoldering slagheap of a uranium mine in Thüringen, Germany. 23 In fact, natural thermophiles have been isolated from the high-temperature zones of dumps and stockpiles where normal, low-temperature bacteria have be used. To date, approximately 30 naturally occurring strains of microorganisms have been screened as being useful in bioleaching.
While it is common to refer to the microorganisms used in bioleaching as being bacteria, strictly speaking, this is not the case since the thermophiles actually fall into another classification of microbiology - the archaea. 24 Thus, iron- and sulfur-oxidizing archaea are currently the microorganisms of choice in the bioleaching of chalcopyrite-containing ores and concentrates.
Three Domains of Life | |
---|---|
Archaea | halophiles and thermophiles |
Bacteria | cyanobacteria and heterotrophic bacteria |
Eukaryota | animals, plants, chromists, fungi, alveolates, rhodophytes, flagellates, basal protists |
At present, the strains of microorganisms used in bioleaching have been cultured from natural strains using methods that are commonly used in other industries, for example, in cheese making, where mesophilic and thermophilic microorganisms are also used. These strains are identical to those found in nature. The only difference is that in some cases they have been selected for rapid growth on the ore or concentrate concerned and to the plant operating conditions. These strains have been characterized, many by DNA sequencing, and listed in the major biological species indices of the world. In the future, perhaps we can expect new, more efficient strains, introduced as a result of genetic manipulation.
Leaching Method 
As indicated above, bioleaching originated by the leaching of low-grade ore dumps, flotation tailings and other waste materials already in place. As the technology has progressed it has moved to more processing-amenable methods: i.e., leaching on a controlled leach pad (heap leaching) (See The Phelps Dodge Mine-for-Leach Project) or in a stirred tank. Each of these methods has a cost and therefore, as shown in Figure 1, the ore-grade and particle size are the controlling factors in making a leaching process choice. 25
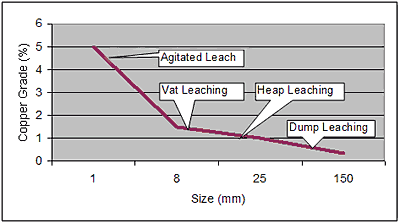
Three of the processes introduced above have made great strides in solving these problems for the extraction of copper from chalcopyrite:
- The GEOCOAT™ Process - A heap leaching process that is capable of bioleaching copper concentrates. It does this by coating the concentrate slurry onto a support rock, or substrate material, and stacking this coated material in a bioxidation heap. The support rock itself may be a low-grade copper ore. The ratio of support rock to concentrate is in the range of 5:1 to 10:1 by weight. The heap is inoculated with thermophilic bacteria and a process leach solution consisting of sulfuric acid, ferric iron and nutrients is applied to the heap. Low-pressure blowers supply air through a system of perforated pipes placed under the heap. The exothermic nature of the oxidation reaction raises the temperature of the heap to at least 50°C (122°F). Typically, oxidation is complete and the copper leached out within 210 days.
- The BioCOP™ Process - Conducted in a stirred reactor containing dilute sulfuric acid into which air is blown, hyperthermophilic microorganisms are used that operate at a temperature between 60°C and 90°C. Limestone is added both to maintain the pH of the solution and to provide carbon dioxide for bacterial growth. Nutrients are added. Copper concentrate is added to the reactor in an amount so as to provide a pregnant liquor containing 30-40 grams per liter (which is reduced to 20-25 grams per liter after washing the leach residue), for feed to the SXEW plant. Leaching of chalcopyrite concentrates is complete within 10 days retention time.
- The BacTech/Mintek Process - Conducted in a series of countercurrent reactors. Two proprietary bioreactors are being tested in Mexico; 1) the Circox™ bioreactor originally developed for the biotreatment of municipal sewage and industrial waste water and licensed from Paques Bio Systems B.V. of the Netherlands. The Circox™ bioreactor uses an airlift to circulate the solids within the reactor, and 2) the BAR™ (BacTech Aerated Reactor). The thermophilic microorganisms used operate at temperatures between 25°C (77°F) and 55°C (131°F). A pH of 0.5 to 2.5 is maintained within the reactor. Carbon dioxide is obtained from the ambient air used to airlift the solids. Nutrients are added to the leach liquor. Retention time is on the order of 30 days.
Costs 
Generally, the capital cost of a bioleaching operation is considerably less, by about 50%, than that of a conventional smelting/refining operation. Quoted operating costs (2002), based on current technology for leaching dumps and in-place ore, are between US$0.18 and US$0.22 per pound of cathode copper, which are competitive with the unit costs of smelting/refining. Heap leaching has the additional cost of mining and transporting to the leach pad. In some operations additional costs are incurred wherein the ROM is crushed and mixed with acid for agglomeration prior to being deposited in the heap. Because of this, as shown in Table 1, direct production costs vary with the grade of the material being leached. 26
Operation | Cost, UScents/lb | |||
---|---|---|---|---|
Grade, % | 0.4 | 0.6 | 0.8 | 1.2 |
Mining | 11.36 | 7.58 | 5.68 | 3.79 |
Leaching | 18.75 | 12.50 | 9.38 | 6.25 |
SX/EW | 14.00 | 14.00 | 14.00 | 14.00 |
Maintenance | 3.20 | 3.20 | 3.20 | 3.20 |
Sales | 2.00 | 2.00 | 2.00 | 2.00 |
Total Cash Cost | 49.31 | 39.38 | 34.26 | 29.24 |
Whether by bioleaching or conventional acid leaching, operating costs for heap leaching for currently operating or planned projects range between US$0.34 and US$0.60. 27
An example of this is Codelco-Chile's operation, Radomiro Tomic, located near Calama, Chile. While a conventional oxide mineral leaching operation, this is the largest heap leaching operation in the world. Radomiro Tomic was built in 1998 at a cost of US$641 million for a design plant capacity of 150,000 metric tons of copper per year. Capital costs at this level of operation were to be US$0.08/lb of copper and operating costs, as shown in Table 2, were estimated at US$0.34/lb of copper. 28 The average ore grade is 0.59% copper and the estimated recovery is 78.2%. The operation was upgraded to 180,000 tons per year before it was completed and to 250,000 tons per year in 2001.
Operation | Cost, UScents/lb | % |
---|---|---|
Mining | 12.52 | 37 |
Leaching | 11.52 | 34 |
SX/EW | 7.81 | 23 |
Maintenance | 1.25 | 4 |
Sales | 0.80 | 2 |
Total Cash Cost | 33.90 | 100 |
Table 3 lists the actual annual operating costs of several conventional and bioleaching projects in 2000. 29
Project | Operating Cost |
---|---|
UScents/lb Cu | |
Morenci (conventional) | 29.5 |
Collahausi (bio) | 30.1 |
Radomiro Tomic (conventional) | 30.2 |
Chuquicomata (conventional) | 39.9 |
El Abra (conventional) | 40.2 |
Chuquicamata SBL (bio) | 50.0 |
Salvador QM (bio) | 60.8 |
As the range these operating costs indicate, to be competitive with conventional smelting, bioleaching will require continued reduction of energy costs which translates into a faster reaction rate and shorter retention times.
Research 
Research is currently underway in a number of organizations to make bioleaching a more efficient and commercially viable process. Because of the long retention times still required, the use of stirred tank technology for copper recovery has been delayed due to its high power requirement for agitating the low pulp densities required. The Chuquicamata SBL, a heap leaching operation of run-of-the-mine low-grade ore, for example, requires a 4-year operating cycle and even then, only about 50% of the contained copper is recovered. 30 Therefore, research is being conducted on improving the kinetics of heap leaching; i.e., reducing the retention time from years to months, and improving the mass transfer in stirred reactors to reduce the retention time from weeks to days. Studies into creating optimum-operating conditions that favor the health of the bacteria are one example. These include: temperature control of the heap or the reactor, providing nutrients (low concentrations of ammonium sulfate, potassium sulfate, and diammonium hydrogen phosphate) and providing sufficient oxygen and carbon dioxide for cell growth.
A research consortium, BioSigma, S.A., has been formed in Chile between Nippon Mining and Metals Co., Ltd. and Codelco in order to conduct and promote research on bioleaching. 31 The new company, which started activities on July 1, 2002, seeks to develop products ranging from relatively simple technologies for optimizing current heap and dump bioleaching processes through better operational control, to the more complex technology such as the identification, characterization and subsequent cloning of proteins and bacteria used in biomining. Special emphasis is being given to developing environmentally sustainable technologies for low-grade ores and for metals recycling.
Conclusions 
Approximately 20% of all copper now produced worldwide is produced by leaching predominantly oxide ores. An undetermined amount of copper is currently recovered from sulfide ores through the aid of naturally occurring microorganisms. If costs can be lowered equivalent to that of the smelting process itself, bioleaching holds promise for the treatment of copper concentrates in situations wherein the capital costs of a smelter are not warranted and/or environmental considerations preclude smelting at that location. With the recently developed technologies that are now under test in demonstration trials and ongoing research into improved technologies, we can safely predict that bioleaching will eventually contribute to an increasing fraction of the copper produced each year.
However, while bioleaching offers several advantages, such as:
- The ability to economically process run-of-the-mine low grade sulfide ores
- The ability to process ores that may not be feasible to be smelted for environmental reasons
it is safe to say that bioleaching will not completely replace smelting for at least three reasons:
- Bioleaching does not recover the precious metals in the ore. The precious metals are often an important component in the profitability of the operation.
- The requirement for the smelter to produce acid for conventional leaching and to supplement bioleaching if the ore body is high in acid consuming minerals. Sulfuric acid is in short supply and expensive to deliver to remote locations.
- Conversely, some ore bodies are not sufficiently high in acid-consuming minerals causing the residual acid that is generated to have to be neutralized external to the leach process - a costly alternative. In such a case, smelting would be the only viable processing means.
References:
- Badilla, Ricardo, BioSigma, SA, Santiago, Chile, personal communication; Leiva S., Hector, Mgr, of Contract Metallurgy, CIMM T&S, S.A., Santiago, Chile, personal communication.
- Leiva S., Hector, loc. cit.
- Pliny the Elder, Natural History: A Selection. Trans. John F. Healy. London: Penguin, 1991.
- Constantinou, G., "Ancient Copper Mining in Cyprus," in Cyprus. Copper and the Sea ed. Anna Marangou. Publication accompanying the Cyprus exhibition at the 1992 Seville Universal Exhibition EXPO 92.
- Hiskey, Brent, The University of Arizona, Tucson, AZ, personal communication.
- Dicinoski, W.B., W.J. Schlitt and V.J. Ambalavaner, "A Global Engineer's Perspective of Copper Leaching, Solvent Extraction and Electrowinning," paper presented at ALTA Copper Hydrometallurgy Forum, Brisbane, Australia, October, 1998.
- Colmer, AR and ME Hinkle, "The Role of Microorganisms in Acid Mine Drainage: a Preliminary Report," Science 106, 1947, p 253 - 256.
- Malouf, E.E. and Prater, J.D. (1961), Role of Bacteria in the Alteration of Sulphide, J. Metals, New York, v13, p353-356.
- Dew, DW and DM Miller, "Copper, nickel and cobalt recovery," U.S. Patent 6,245,125, June 12, 2001.
- Spencer, PA, JR Budden, J Barrett, MN Hughs and R K Poole, "Oxidation of metal sulfides using thermotolerant bacteria," U.S. Patent 5,429,659, July 4, 1995.
- Hutchins, SR, MS Davidson, JA Brierley and CL Brierley, "Thermophilic microbial treatment of precious metal ores," U.S. Patent 4,729,788, March 8, 1988.
- Kohr, WJ, "Nonstirred bioreactor for processing refractory sulfide concentrates and method for operating same," U.S. Patent 6,107,065, August 22, 2000.
- Kohr, WJ, "High Temperature heap bioleaching process," U.S. Patent 6,110,253, August 29, 2000.
- MINTEK is a South African minerals research and technology organization affiliated with the government of South Africa. It receives its funding both from government and industry.
- BacTech Press Release, October 2, 2001.
- Anon, "Bioleaching Moves forward," Mining J. May 31, 2002, p 392.
- Leiva, Hectro, CIMM, Santiago, Chile, personal communication
- Brierley, CL and JA Brierley, "Present and future applications of biohydrometallurgy," Hydrometallurgy 59, 2001, 233-239.
- Brierley, CL and JA Brierley, "A chemoautotrotpic and thermophilic microorganism isolated from an acid hot spring," Can. J. Microbiol. 19, 1973, pp 183 - 188.
- Segerer, A., A Neuner, JK Kristjansson & KO Stetter, "Acidianus infernus gen. nov., sp. nov., and Acidianus brierleyi comb. nov.: Facultatively aerobic, extremely acidophilic thermophilic sulfur-metabolizing archaebacteria.," Int. J. Syst. Bacteriol. 36, 1986, pp 559-564.
- Huber, G and KO Stetter, "Sulfolobus metallicus, sp. nov., a novel strictly chemolithoautotrophic thermophilic archaeal species of metal-mobilizers," Syst. Appl. Microbiol. 17, 1991, pp 372 - 378.
- Huber, G, C Spinnler, A Gambacorta and KO Stetter, "Metallosphaera sedula gen. nov. and sp. nov. represents a new genus of aerobic, metal-mobilizing, thermoacidophilic archaebacteria," Syst. Appl. Microbiol. 12, 1989, pp 37 - 47.
- Fuchs T, H Huber, K Teiner, S Burggraf and KO Stetter, "Metallosphaera prunae," System Applied Microbiology 18, 1995 pp 560-566.
- Woese, CR and G E Fox, "Phylogenetic structure of the prokaryotic domain: The primary kingdoms," Proc. Natl. Acad. Sci. USA 74, 1977, pp 5088-5090.
- Badilla, Ricardo, loc cit
- Badilla, Ricardo, loc cit
- Long, KR and Singer, DA, "A simplified Filter for Open-Pit Mining and Heap Leaching Recovery of Copper in the United States, Open File Report 01-218, United States Department of the Interior, Geological Survey, 2001, 21p.
- Badilla, Ricardo, loc cit
- Badilla, Ricardo, loc cit
- Codelco.
- BioSigma S.A., Avda Nueva de Lyon 072, Of 1802, Providencia, Santiago, Chile; Phone 56.2.333 1922 fax 56.2.335 8140; email: [email protected].
Also in this Issue:
- Producing Copper Nature's Way: Bioleaching