Contents
- Introduction
- Engineering Applications
- Chemical Compositions
- Mechanical Properties
- Physical Properties
- Availability
- Fatigue Properties
- Other Principal Properties of High Strength Cu-Ni alloys
- Machining and Fabrication
- Useful papers
INTRODUCTION
The principle high strength Cu-Ni alloys result from the fact that the addition of aluminium to a Cu-Ni binary alloy increases the strength through the formation of age-hardening precipitates. This effect has been known since the 1930’s, and a number of alloys have been produced based on the Cu-Ni-Al system. The first commercial aluminium-containing Cu-Ni alloy was HIDURAX SPECIAL (Cu-15Ni-3Al), developed in the 1940’s to meet a requirement for a high strength corrosion resistant alloy in naval applications. This alloy was later given the generic designations DIN 2.1504, UNS C72400 and the manufacturing supply code DTD 900/4805.
The basic strengthening process in Cu-Ni-Al alloys is achieved through the formation of nanometre-scale Ni3Al (γ’) phase precipitates. It has been found that the effectiveness of this phase in increasing the mechanical properties can be improved through the addition of other elements. For instance, precise crystallographic measurements of the microstructure have proved that iron, manganese and niobium additions control the crystallographic structure of the fine Ni3Al precipitates and increase their efficiency in producing alloy strengthening.
The precipitation-hardened high strength Cu-Ni alloys have a unique set of properties which make them particularly suitable for exacting marine applications. Later developments of the original commercial Cu-Ni-Al material have produced NES 835 (also denoted by DOD-C-24676 and UNS C72420) and Cu-Ni-Mn-Al-Nb, the proprietary alloy MARINEL (Trade Mark of Langley Alloys Ltd). The main features of these materials is that they display excellent all-round corrosion resistance, immunity to hydrogen embrittlement, high impingement resistance, good resistance to stress corrosion cracking, excellent anti-galling characteristics, a high modulus of elasticity, low magnetic properties, anti-biofouling characteristics and ease of machining.
Back to TopENGINEERING APPLICATIONS
High strength Cu-Ni alloys, with their unique set of properties, enjoy a wide variety of engineering applications. These can be summarised by market sector in the following way:
- Oil and Gas Industry Bolting for risers, subsea manifolds, heat exchangers, caissons and splash zone, as well as for down-hole equipment. Stab plate connectors, actuator components, shafts for pumps & valves, valve stems and subsea clamps.
- Defence Applications Bolting for ships and submarines. Towing and winch components. Components in sonar equipment. Propeller shafts, drive bushes and bearings.
- Aerospace Components in head-up displays. Electrical connectors and bearings.
- Automotive Valve components, connector rods and cylinder liners.
CHEMICAL COMPOSITIONS (wt%)
UNS C72400 | DIN 2.1504 | UNS C72420 | DGS 229 | DOD-C-24676 | DEF STAN 02-835 (NES 835) | MARINEL 220 Cu-Ni-Mn-Al-Nb | |
---|---|---|---|---|---|---|---|
Aluminium | 1.5 – 2.5 | 2.00 – 3.00 | 1.0-2.0 | 1.4 – 2.8 | 1.0-2.0 | 1.00-2.00 | 1.60 - 2.20 |
Iron | 0.10 max | 1.50 max | 0.7-1.2 | 3.5 – 6.0 | 0.7-1.2 | 0.70-1.20 | 0.65 – 0.85 |
Nickel | 11.0 – 15.0 | 13.00 – 16.00 | 13.5-16.5 | 9 – 11.5 | 13.5-16.5 | 13.50-16.50 | 18.00 - 25.00 |
Manganese | 1.0 max | 1.0 max | 3.5-5.5 | 11 -15 | 3.5-5.5 | 3.50-5.50 | 4.00 - 5.60 |
Chromium | - | - | 0.50 max | 0.5 max | 0.5 max | 0.50 max | 0.30 – 0.50 |
Niobium | - | - | - | - | - | - | 0.55 – 0.90 |
Copper | Remai-nder | Remai-nder | Remai-nder | Remai-nder | Remai-nder | Remai-nder | Remai-nder |
Lead | 0.05 max | - | 0.02 max | 0.01 max | 0.02 max | 0.02 max | 0.02 Max |
Tin | 0.05 max | - | 0.10 max | 0.10 max | 0.10 max | 0.10 max | 0.10 Max |
Zinc | 0.50 max | - | 0.20 max | 0.20 max | 0.20 max | 0.20 max | 0.20 Max |
Silicon | - | 0.3 Max | 0.15 max | 0.15 max | 0.15 max | 0.15 max | 0.15 Max |
Magnesium | 0.05 – 0.40 | 0.1 Max | 0.05 max | 0.10 max | 0.05 max | 0.05 max | 0.05 Max |
Sulphur | - | - | 0.15 max | 0.015 max | 0.15 max | 0.15 max | 0.15 Max |
Carbon | - | - | 0.05 max | 0.05 max | 0.05 max | 0.05 max | 0.05 Max |
Phosphorus | - | - | 0.01 max | - | 0.01 max | 0.01 Max | 0.01 Max |
Impurities | - | 0.50 Max | - | - | 0.30 max | 0.30 max | 0.30 Max |
MECHANICAL PROPERTIES
DTD 900/4805 (HIDURAX SPECIAL/HIDURON 130)
Section Size | Up to 65mm | Over 65mm |
---|---|---|
Tensile Strength, N/mm2 | 850 min | 770 min |
0.2% Proof Stress, N/mm2 | 630 min | 555 min |
Elongation on 5.65/So, % | 10 min | 10 min |
Brinell Hardness Number | 240 min | 229 min |
DGS 229
Tensile Strength, N/mm2 | 463 min |
0.5% Proof Stress, N/mm2 | 262 min |
Elongation on 5.65/So, % | 30 min |
Izod, J | 47 min |
DEF STAN 02 835 (formerly NES 835)
Section Size | Minimum Tensile Strength N/mm2 | Minimum 0.2% Proof Stress N/mm2 | Minimum Elongation On 5.65 /So % | Minimum Izod Impact Joules |
---|---|---|---|---|
Rods & sections up to and including 125mm | 725 | 430 | 18 | 40 |
Rods and sections over125mm and forgings | 710 | 400 | 18 | 40 |
DOD-C-24676
Section Size | Minimum Tensile Strength psi | Minimum 0.2% Proof Stress psi | Minimum Elongation On 4 /So % |
---|---|---|---|
Not Specified | 103,000 | 58,000 | 18 |
Cu-Ni-Mn-Al-Nb (MARINEL)
Section Size | Minimum Tensile Strength N/mm2 | Minimum 0.2% Proof Stress N/mm2 | Minimum Elongation On 5.65 /So % | Brinell Hardness HB | Minimum Izod Impact Joules |
---|---|---|---|---|---|
Ruling sections up to & including 4” (101.6mm) | 870 | 700 | 15 | 240-320 | 18 |
Rods & sections over 4” (101.6mm) & up to & including 6” (152.4mm) | 870 | 650 | 15 | 240-320 | 18 |
Rods and sections over 6” (152.4mm) and forgings | 840 | 650 | 12 | 240-320 | 27 |
From the above tables, it can be seen that copper-nickel alloys are available with high strengths and, in some cases, equivalent strengths to carbon steel. Additionally, it should be noted that, unlike many materials, impact properties of high strength copper-nickels are fully retained at sub-zero temperatures down to -196°C.
Back to TopPHYSICAL PROPERTIES
Physical Properties: | |
---|---|
Melting range °C | 1030°C - 1085°C |
Coefficient of thermal expansion (20-300°C).°K-1 | 16.0 x 10-6 |
Thermal conductivity at 20°C, watt/metre kelvin | 25 |
Specific heat at 20°C, joule/kilogram kelvin | 435 |
Electrical resistivity at 20°C, ohm mm2/ m | 0.35 |
Electrical conductivity at 20°C, m/ohm mm2 | 2.85 |
Magnetic permeability | <1.01 |
Density at 20°C, g/cm3 | 8.53 |
AVAILABILITY
Specification | Trade Marks | Trademark Holder | Forms Available |
---|---|---|---|
DTD 900/4805 | HIDURAX SPECIAL | Langley Alloys Ltd, UK | Bar, Forgings |
HIDURON 130 | Langley Alloys Ltd, UK | Bar, Forgings | |
DIN 2.1504 | NIBRON SPECIAL | Columbia Metals, UK | Bar, Forgings |
HIDURON 130 | Langley Alloys Ltd, UK | Bar, Forgings | |
DGS 229 | HIDURON 501 | Langley Alloys Ltd, UK | Castings |
DOD-C-24676 | HIDURON 191 | Langley Alloys Ltd, UK | Bar, Forgings |
DEF STAN 02-835 (formerly NES 835) | HIDURON 191 | Langley Alloys Ltd, UK | Bar, Forgings |
Cu-Ni-Mn-Al-Nb | MARINEL 220 | Langley Alloys Ltd, UK | Bar, Forgings |
FATIGUE PROPERTIES
Results for the fatigue limit in air for NES 835, DTD 900/4805 and Cu-Ni-Mn-Al-Nb are given below
Specification | Fatigue Limit (MPa) |
---|---|
NES 835 | 230 |
DTD 900/4805 | 270 |
Cu-Ni-Mn-Al-Nb | 310 |
Cu-Ni-Mn-Al-Nb possesses a rotating bend fatigue S-N curve as shown in the graph below. No noticeable difference is found between testing in air and testing in artificial seawater, producing the following results:
Fatigue limit in air (108 cycles) | - 310 N/mm2 |
Fatigue limit in artificial seawater (108 cycles) | - 310 N/mm2 |
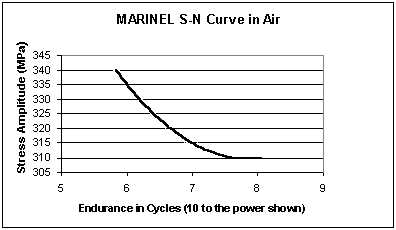
OTHER PRINCIPAL PROPERTIES OF HIGH STRENGTH CU-NI ALLOYS
Resistance to Hydrogen Embrittlement
Most high strength engineering alloys suffer a reduction in mechanical properties when they interact with hydrogen. This phenomenon is known as hydrogen embrittlement (HE) and it is a particular concern when cathodic protection is applied to protect an immersed steel structure or when dissimilar metals are coupled in seawater. These situations will stimulate the release of nascent hydrogen which can penetrate the metal surface and act to reduce ductility. Hydrogen embrittlement can occur by a number of different mechanisms, depending on the alloy type concerned. Zirconium and titanium alloys undergo embrittlement due to the formation of internal hydrides within the material. Steel and nickel alloys lose ductility through the retention of hydrogen at internal discontinuities within the microstructure. Thus, if bolts or other components of these materials are under tension under cathodic protection conditions in seawater, material failure through HE can occur relatively rapidly.
During the 1980’s, hydrogen embrittlement was experienced as a particular problem in offshore nickel alloy bolting and the oil & gas industry sought a solution by sponsoring extensive testing programmes on a number of high strength materials. Most high strength alloys have been tested including nickel alloys, stainless steels, carbon steel and the high strength Cu-Ni alloys NES 835 and Cu-Ni-Mn-Al-Nb. Of all the materials tested, Cu-Ni-Mn-Al-Nb and NES 835 have been the only materials to consistently show no effects of hydrogen embrittlement, as shown by the table below, which is taken from work carried out at the BNF Laboratories, UK in 1990.
U.T.S (N/mm2) | %Elong | Reduction of area (%) | ||
---|---|---|---|---|
Cu-Ni-Mn-Al-Nb | Air | 1005 | 14 | 33 |
After CP charging | 1004 | 13 | 31 | |
K-500 Nickel Alloy | Air | 1139 | 27 | 46 |
After CP charging | 1101 | 15 | 18 | |
625 Nickel Alloy | Air | 1146 | 44 | 54 |
After CP charging | 1150 | 44 | 47 | |
925 Nickel Alloy | Air | 1216 | 28 | 48 |
After CP charging | 1215 | 25 | 30 |
The reasons why high strength Cu-Ni alloys are resistant to HE have been proposed to be due to the observation that hydrogen is less able to enter the material and to be retained by internal discontinuities than alloys which are known to be susceptible to HE. Thus, unlike steel and nickel alloys, where hydrogen entry is rapid and accumulation of hydrogen at particles and precipitates is considered to promote crack growth, high strength Cu-Ni alloys do not suffer hydrogen embrittlement.
Abuse Tolerance and Resistance to Stress Corrosion Cracking
Cu-Ni alloys are noted in the literature for being much more resistant to stress corrosion cracking (SCC) than brasses and aluminium bronzes. Work which has been carried out on the stress corrosion cracking behaviour of 90-10 Cu-Ni’s with additions of iron in ammonia and this has shown that resistance to SCC is produced by restricting the iron content to below 1% and retaining the iron in solution. If iron is above a 1% level and out of solution, intergranular stress corrosion has been observed to occur. MARINEL 220, which represents the latest version of the alloy (with an iron content controlled to be less than 0.85%) shows resistance to environmental effects and significant abuse tolerance.
C-ring stress corrosion testing has been carried out after the application of adhesives, coatings and locking media which are often used on bolting materials in service. The overall result is that MARINEL 220 is suitable to use both in a coated or uncoated condition without the risk of experiencing environment-assisted degradation of properties.
Resistance to Corrosion and Stress Corrosion Cracking in Hydrogen Sulphide Environments
Scanning electron microscopy of Cu-Ni-Mn-Al-Nb, carried out after exposure in acidified hydrogen sulphide solution at room temperature, reveals that the aluminium content of the material produces an oxide layer which gives the alloy a considerable degree of corrosion protection. This would explain its superior behaviour over standard Cu-Ni alloys in sulphide environments.
It has been demonstrated that Cu-Ni-Mn-Al-Nb successfully resists sulphide stress corrosion and is well able to satisfy the requirements of NACE MR-01-77.
Anti-Galling Properties
The resistance to pickup by high strength Cu-Ni alloys is very good, the alloys generally running against nickel aluminium bronze and stainless steels. DEF STAN 02 835 (formerly NES 835), DOD-C-24676 and Cu-Ni-Mn-Al-Nb have demonstrated superior anti-galling properties when used in bearing and actuator applications.
General Corrosion Resistance
The general corrosion rates of the high strength Cu-Ni alloys have been determined by immersion in seawater off the south coast of Britain and can be compared to other corrosion resistant alloys which have been investigated under similar conditions. A chart showing the comparative seawater corrosion rates for different materials is given below and demonstrates the low corrosion rates of the two high strength Cu-Ni alloys DEF STAN 02 835 (formerly NES 835) and Cu-Ni-Mn-Al-Nb. The corrosion rates of Cu-Ni-Al alloys are observed to reduce with time, as initially a dark copper oxide-rich layer is formed which acts to stifle further corrosion of the material.
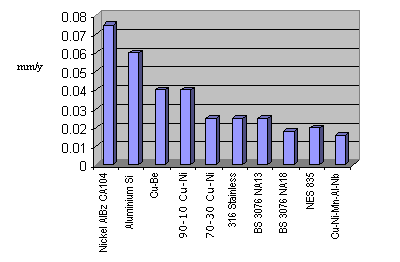
Biofouling Resistance
High strength Cu-Ni alloys are able to effectively resist the deposition and build up of marine organisms, making them ideal in terms of inspection and maintenance when employed in submerged seawater service.
Galvanic Compatibility
Laboratory tests and application experience has demonstrated that the aluminium-hardened Cu-Ni alloys are galvanically compatible with most copper based alloys, including 70-30 Cu-Ni and nickel aluminium bronze. However, where cathodic protection is not applied, it is good design practice to minimise the danger of galvanic corrosion by ensuring the more noble components (cathodic) are of smaller surface area than the less noble material (anodic).
The table below shows the results of galvanic coupling tests carried out at the LaQue Center for Corrosion Technology, USA and Langstone Harbour, UK on common marine alloys coupled with S32550 superduplex stainless steel. Visually, no enhanced attack on the Cu-Ni alloys under the applied crevice conditions was observed. Generally, when dissimilar metals are present, Cu-Ni-Al alloys are more noble than carbon steel thus, if these alloys are used for bolting carbon steel, any hydrogen liberated by the couple will not adversely affect the Cu-Ni bolts.
ALLOY | Average Seawater Temperature (°C) | Rest Potential (uncoupled) [mV vs SCE] | DEPTH OF ATTACK (mm) | |
---|---|---|---|---|
Uncoupled | Coupled | |||
Nickel Aluminium Bronze | 30 | -180 | 0.02 | 0.12 |
70-30 Ni-Cu (Alloy 400) | 30 | -140 | >0.13 | >0.26 |
90-10 Cu-Ni | 30 | -250 | 0.03 | 0.44 |
316 Stainless Steel | 30 | -450 | >0.20 | >0.74 |
DEF STAN 02 835 (formerly NES 835) | 30 | -100 | 0.02 | 0.04 |
Cu-Ni-Mn-Al-Nb | 12 | -190 | 0.018 | 0.035 |
UNS S32550 | 12 and 30 | +50 | Not applicable | Not applicable |
High resistance to Erosion Corrosion
Jet impingement tests have been carried out on high strength Cu-Ni-Al alloys in aerated seawater flowing at 9.3 m/s for 28 days and show that these materials have resistance to impingement attack. Their corrosion properties in this case are similar to cast nickel aluminium bronze and superior to wrought iron-containing 70-30 and 90-10 Cu-Ni alloys. Results are given in the table below.
ALLOY | EROSION PIT DEPTH (μm) | |
---|---|---|
5°C | 10°C | |
70-30 Cu-Ni | 70 | 50 |
90-10 Cu-Ni | 12 | 10 |
High Strength Cu-Ni alloys | 4 | 2 |
Cavitation erosion tests in sodium chloride also show the superiority of Cu-Ni-Mn-Al-Nb over other marine corrosion resistant materials, as shown below.
Results of cavitation erosion tests carried out through agitation at 20Hz in 3% Sodium Chloride
ALLOY | CAVITATION EROSION RATE (mm3/h) |
---|---|
90-10 Cu-Ni | 1.5 |
70-30 Cu-Ni | 1.9 |
316 Stainless steel | 1.7 |
Alloy K-500 | 1.2 |
DEF STAN 02 835 (formerly NES 835) | 1.8 |
Cu-Ni-Mn-Al-Nb | 1.0 |
MACHINING AND FABRICATION
Welding
Welding can generally be carried out, either by the use of matched welding consumables (where available) or nickel aluminium bronze welding consumables. In the latter case, the strength properties of the weld region will be probably lower than the parent material.
Brazing & Soldering
High strength Cu-Ni-Al can be brazed by the same procedures as for the aluminium bronzes using special fluoride containing fluxes and silver brazing alloys with low melting point (about 650°C). Soft soldering can also be carried out by similar procedures to those employed for the aluminium bronzes using a flux of phosphoric acid solution with a minimum specific gravity of 1.75.
Machining
High strength Cu-Ni alloys can be easily machined to give an extremely good finish, using methods normally employed with high strength aluminium bronzes. Thread rolling in the production of fasteners may be employed and a relatively small increase in hardness in the thread form is encountered.
Dimensional stability is good, and although normally no stress relief is required, in special cases where dimensional tolerances are critical it may be desirable to heat treat for I to 2 hours in an air circulating furnace at 300°C followed by air cool.
Tooling should be carbide tipped with water soluble lubricant applied.
Turning: | Roughing Speed | 120-80 mpmin |
---|---|---|
Roughing Feed | 0.4-0.75 mmpr | |
Finishing Speed | 150-300 mpmin | |
Finishing Feed | 0.15-0.4 mmpr | |
Drilling: | Speed | 25-75 mpmin |
Feed | 0.075-0.5 mmpr | |
Reaming: | Speed | Up to 60 mpmin |
Tapping: | Speed | 20-45 mpmin |
Feed | 0.2-1.0 mmpr | |
Milling: | Speed | 45-60 mpmin |
References
- An improved performance super high strength copper nickel alloy for use in offshore oil and gas and other marine environments, Tuck, C. D. S., N. Hort, and B. L. Mordike, , Corrosion 99, San Antonio, TX, NACE, .
- High Strength Copper Nickel – Optimisation of Mechanical Strength and Marine Corrosion Resistance for Use in Naval Architecture and Offshore Oil & Gas, C D S Tuck, K C Bendall, G W J Radford, S A Campbell and R J Grylls, Paper No. 78, Corrosion /96, Houston, NACE, .
- High Strength Cupronickel for Marine Service, C A Clark and P Guha, Br. Corros. J., Anti-Corrosion Methods and Materials, Vol. 36, 8, pp 4-6,12, , .
- Hydrogen embrittlement resistance of ultrahigh strength cupronickel alloy: Effects of exposure to gaseous hydrogen environment on fatigue resistance, Tuck, C. D. S., Zeng Xianghua, and D. E. J. Talbot, British Corrosion Journal, Vol. 29, 1, pp 70-74, , Institute of Materials, .
- Hydrogen Ingress Into Copper-Nickel Alloys, B G Pound, Corrosion (USA), Vol. 50, 4, pp 301-307, , NACE, .
- Observation of a high-strength cupronickel alloy using electron microscopy, Grylls, R. J., Tuck, C. D. S., & Loretto, M. H., Institute of Physics Conference Series, No. 147, Section 11, pp 459-462, , .
- Testing of the effects of hydrogen on various high strength alloys for subsea application, Bardal, E., Eggen, T. G., & Skar, J. I., pp 6, , Associazione Italiana diMetallurgia, .